Berikut merupakan kutipan ilmiah kedokteran yang sangat bermanfaat untuk diketahui sehingga disusun dan digunakan sebagai referensi pribadi. Dengan hormat, saya mohon izin disampaikan kepada narasumber. Semoga Bermanfaat.
Perpustakaan Keluarga : Helmut Todo Tua Simamora dan dr. Olga, Y.V Hutapea
Heart rate is the speed of the heartbeat measured by the number of contractions of the heart per minute (bpm). The heart rate can vary according to the body's physical needs, including the need to absorb oxygen and excrete carbon dioxide. It is usually equal or close to the pulse measured at any peripheral point. Activities that can provoke change include physical exercise, sleep, anxiety, stress, illness, ingesting, and drugs.
The normal resting adult human heart rate ranges from 60–100 bpm.[1] Tachycardia is a fast heart rate, defined as above 100 bpm at rest.[2] Bradycardia is a slow heart rate, defined as below 60 bpm at rest. During sleep a slow heartbeat with rates around 40–50 BPM is common and is considered normal. When the heart is not beating in a regular pattern, this is referred to as an arrhythmia. These abnormalities of heart rate sometimes indicate disease.[3]

Physiology
While heart rhythm is regulated entirely by the sinoatrial node under normal conditions, heart rate is regulated by sympathetic and parasympathetic input to the sinoatrial node. The accelerans nerveprovides sympathetic input to the heart by releasing norepinephrine onto the cells of the sinoatrial node (SA node), and the vagus nerve provides parasympathetic input to the heart by releasing acetylcholineonto sinoatrial node cells. Therefore, stimulation of the accelerans nerve inNormal heart sounds
Normal heart sounds as heard with a stethoscope
Problems playing this file? See media help.creases heart rate, while stimulation of the vagus nerve decreases it.[4]
Due to individuals having a constant blood volume, one of the physiological ways to deliver more oxygen to an organ is to increase heart rate to permit blood to pass by the organ more often.[3] Normal resting heart rates range from 60–100 bpm. Bradycardia is defined as a resting heart rate below 60 bpm. However, heart rates from 50 to 60 bpm are common among healthy people and do not necessarily require special attention. Tachycardia is defined as a resting heart rate above 100 bpm, though persistent rest rates between 80–100 bpm, mainly if they are present during sleep, may be signs of hyperthyroidism or anemia (see below).[3]
- Central nervous system stimulants such as substituted amphetamines increase heart rate.
- Central nervous system depressants or sedatives decrease the heart rate (apart from some particularly strange ones with equally strange effects, such as ketamine which can cause - amongst many other things - stimulant-like effects such as tachycardia).
There are many ways in which the heart rate speeds up or slows down. Most involve stimulant-like endorphins and hormones being released in the brain, many of which are those that are 'forced'/'enticed' out by the ingestion and processing of drugs.
This section discusses target heart rates for healthy persons and are inappropriately high for most persons with coronary artery disease.[5]

Influences from the central nervous system
Cardiovascular centres
The heart rate is rhythmically generated by the sinoatrial node. It is also influenced by central factors through sympathetic and parasympathetic nerves.[6] Nervous influence over the heartrate is centralized within the two paired cardiovascular centres of the medulla oblongata. The cardioaccelerator regions stimulate activity via sympathetic stimulation of the cardioaccelerator nerves, and the cardioinhibitory centers decrease heart activity via parasympathetic stimulation as one component of the vagus nerve. During rest, both centers provide slight stimulation to the heart, contributing to autonomic tone. This is a similar concept to tone in skeletal muscles. Normally, vagal stimulation predominates as, left unregulated, the SA node would initiate a sinus rhythm of approximately 100 bpm.[7]
Both sympathetic and parasympathetic stimuli flow through the paired cardiac plexus near the base of the heart. The cardioaccelerator center also sends additional fibers, forming the cardiac nerves via sympathetic ganglia (the cervical ganglia plus superior thoracic ganglia T1–T4) to both the SA and AV nodes, plus additional fibers to the atria and ventricles. The ventricles are more richly innervated by sympathetic fibers than parasympathetic fibers. Sympathetic stimulation causes the release of the neurotransmitter norepinephrine (also known as noradrenaline) at the neuromuscular junction of the cardiac nerves. This shortens the repolarization period, thus speeding the rate of depolarization and contraction, which results in an increased heartrate. It opens chemical or ligand-gated sodium and calcium ion channels, allowing an influx of positively charged ions.[7]
Norepinephrine binds to the beta–1 receptor. High blood pressure medications are used to block these receptors and so reduce the heart rate.[7]
Parasympathetic stimulation originates from the cardioinhibitory region with impulses traveling via the vagus nerve (cranial nerve X). The vagus nerve sends branches to both the SA and AV nodes, and to portions of both the atria and ventricles. Parasympathetic stimulation releases the neurotransmitter acetylcholine (ACh) at the neuromuscular junction. ACh slows HR by opening chemical- or ligand-gated potassium ion channels to slow the rate of spontaneous depolarization, which extends repolarization and increases the time before the next spontaneous depolarization occurs. Without any nervous stimulation, the SA node would establish a sinus rhythm of approximately 100 bpm. Since resting rates are considerably less than this, it becomes evident that parasympathetic stimulation normally slows HR. This is similar to an individual driving a car with one foot on the brake pedal. To speed up, one need merely remove one’s foot from the brake and let the engine increase speed. In the case of the heart, decreasing parasympathetic stimulation decreases the release of ACh, which allows HR to increase up to approximately 100 bpm. Any increases beyond this rate would require sympathetic stimulation.[7]
Input to the cardiovascular centres[edit]
The cardiovascular centres receive input from a series of visceral receptors with impulses traveling through visceral sensory fibers within the vagus and sympathetic nerves via the cardiac plexus. Among these receptors are various proprioreceptors,baroreceptors, and chemoreceptors, plus stimuli from the limbic system which normally enable the precise regulation of heart function, via cardiac reflexes. Increased physical activity results in increased rates of firing by various proprioreceptors located in muscles, joint capsules, and tendons. The cardiovascular centres monitor these increased rates of firing, suppressing parasympathetic stimulation or increasing sympathetic stimulation as needed in order to increase blood flow.[7]
Similarly, baroreceptors are stretch receptors located in the aortic sinus, carotid bodies, the venae cavae, and other locations, including pulmonary vessels and the right side of the heart itself. Rates of firing from the baroreceptors represent blood pressure, level of physical activity, and the relative distribution of blood. The cardiac centers monitor baroreceptor firing to maintain cardiac homeostasis, a mechanism called the baroreceptor reflex. With increased pressure and stretch, the rate of baroreceptor firing increases, and the cardiac centers decrease sympathetic stimulation and increase parasympathetic stimulation. As pressure and stretch decrease, the rate of baroreceptor firing decreases, and the cardiac centers increase sympathetic stimulation and decrease parasympathetic stimulation.[7]
There is a similar reflex, called the atrial reflex or Bainbridge reflex, associated with varying rates of blood flow to the atria. Increased venous return stretches the walls of the atria where specialized baroreceptors are located. However, as the atrial baroreceptors increase their rate of firing and as they stretch due to the increased blood pressure, the cardiac center responds by increasing sympathetic stimulation and inhibiting parasympathetic stimulation to increase HR. The opposite is also true.[7]
Increased metabolic byproducts associated with increased activity, such as carbon dioxide, hydrogen ions, and lactic acid, plus falling oxygen levels, are detected by a suite of chemoreceptors innervated by the glossopharyngeal and vagus nerves. These chemoreceptors provide feedback to the cardiovascular centers about the need for increased or decreased blood flow, based on the relative levels of these substances.[7]
The limbic system can also significantly impact HR related to emotional state. During periods of stress, it is not unusual to identify higher than normal HRs, often accompanied by a surge in the stress hormone cortisol. Individuals experiencing extreme anxiety may manifest panic attacks with symptoms that resemble those of heart attacks. These events are typically transient and treatable. Meditation techniques have been developed to ease anxiety and have been shown to lower HR effectively. Doing simple deep and slow breathing exercises with one’s eyes closed can also significantly reduce this anxiety and HR.[7]
Factors influencing heart rate
| ||||||||||||||||||||||||||
|
Using a combination of autorhythmicity and innervation, the cardiovascular center is able to provide relatively precise control over the heart rate, but other factors can impact on this. These include hormones, notably epinephrine, norepinephrine, and thyroid hormones; levels of various ions including calcium, potassium, and sodium; body temperature; hypoxia; and pH balance.[7]
Epinephrine and norepinephrine
The catecholamines, epinephrine and norepinephrine, secreted by the adrenal medulla form one component of the extended fight-or-flight mechanism. The other component is sympathetic stimulation. Epinephrine and norepinephrine have similar effects: binding to the beta-1 adrenergic receptors, and opening sodium and calcium ion chemical- or ligand-gated channels. The rate of depolarization is increased by this additional influx of positively charged ions, so the threshold is reached more quickly and the period of repolarization is shortened. However, massive releases of these hormones coupled with sympathetic stimulation may actually lead to arrhythmias. There is no parasympathetic stimulation to the adrenal medulla.[7]
Thyroid hormones
In general, increased levels of the thyroid hormones (thyroxine(T4) and triiodothyronine (T3)), increase the heart rate; excessive levels can trigger tachycardia. The impact of thyroid hormones is typically of a much longer duration than that of the catecholamines. The physiologically active form of triiodothyronine, has been shown to directly enter cardiomyocytes and alter activity at the level of the genome.[clarification needed] It also impacts the beta adrenergic response similar to epinephrine and norepinephrine.[7]
Calcium
Calcium ion levels greatly impact on heartrate and contractility; increased levels cause an increase in both. High levels of calcium ions result in (hypercalcemia) and excessive levels can induce cardiac arrest. Drugs known as calcium channel blockers slow HR by binding to these channels and blocking or slowing the inward movement of calcium ions.[7]
Caffeine and nicotine
Factors decreasing heart rate[edit]Caffeine and nicotine are both stimulants of the nervous system and of the cardiac centres causing an increased heart rate. Caffeine works by increasing the rates of depolarization at the SA node, whereas nicotine stimulates the activity of the sympathetic neurons that deliver impulses to the heart.[7] Both stimulants are legal and unregulated, and are known to be very addictive.[7]
The heart rate can be slowed by altered sodium and potassium levels, hypoxia, acidosis, alkalosis, and hypothermia. The relationship between electrolytes and HR is complex, but maintaining electrolyte balance is critical to the normal wave of depolarization. Of the two ions, potassium has the greater clinical significance. Initially, both hyponatremia (low sodium levels) and hypernatremia (high sodium levels) may lead to tachycardia. Severely high hypernatremia may lead to fibrillation, which may cause CO to cease. Severe hyponatremia leads to both bradycardia and other arrhythmias. Hypokalemia (low potassium levels) also leads to arrhythmias, whereas hyperkalemia (high potassium levels) causes the heart to become weak and flaccid, and ultimately to fail.[7]
Heart muscle relies exclusively on aerobic metabolism for energy. Hypoxia (an insufficient supply of oxygen) leads to decreasing HRs, since metabolic reactions fueling heart contraction are restricted.[7]
Acidosis is a condition in which excess hydrogen ions are present, and the patient’s blood expresses a low pH value. Alkalosis is a condition in which there are too few hydrogen ions, and the patient’s blood has an elevated pH. Normal blood pH falls in the range of 7.35–7.45, so a number lower than this range represents acidosis and a higher number represents alkalosis. Enzymes, being the regulators or catalysts of virtually all biochemical reactions - are sensitive to pH and will change shape slightly with values outside their normal range. These variations in pH and accompanying slight physical changes to the active site on the enzyme decrease the rate of formation of the enzyme-substrate complex, subsequently decreasing the rate of many enzymatic reactions, which can have complex effects on HR. Severe changes in pH will lead to denaturation of the enzyme.[7]
The last variable is body temperature. Elevated body temperature is called hyperthermia, and suppressed body temperature is called hypothermia. Slight hyperthermia results in increasing HR and strength of contraction. Hypothermia slows the rate and strength of heart contractions. This distinct slowing of the heart is one component of the larger diving reflex that diverts blood to essential organs while submerged. If sufficiently chilled, the heart will stop beating, a technique that may be employed during open heart surgery. In this case, the patient’s blood is normally diverted to an artificial heart-lung machine to maintain the body’s blood supply and gas exchange until the surgery is complete, and sinus rhythm can be restored. Excessive hyperthermia and hypothermia will both result in death, as enzymes drive the body systems to cease normal function, beginning with the central nervous system.[7]
Heart rates in different circumstances
Abbreviation | Meaning |
---|---|
bpm | beats per minute |
HR | heart rate |
THR | target heart rate |
HRmax | Maximum heart rate |
HRrest | Resting heart rate |
Heart rate is not a stable value and it increases or decreases in response to the body's need in a way to maintain an equilibrium (basal metabolic rate) between requirement and delivery of oxygen and nutrients. The normal SA node firing rate is affected by autonomic nervous system activity: sympathetic stimulation increases and parasympathetic stimulationdecreases the firing rate.[8] A number of different metrics are used to describe heart rate.
Resting heart rate
The basal or resting heart rate (HRrest) is defined as the heart rate when a person is awake, in a neutrally temperateenvironment, and has not undergone any recent exertion or stimulation, such as stress or surprise. The typical resting heart rate in adults is 60–100 beats per minute (bpm). For endurance athletes at the elite level, it is not unusual to have a resting heart rate between 33 and 50 bpm.[citation needed] This is the firing rate of the heart sinoatrial node, where the faster heart pacemaker cells driving the self-generated rhythmic firing and responsible for the cardiac muscle automaticity[9] are located.
Target heart rate
For healthy people, the Target Heart Rate or Training Heart Rate (THR) is a desired range of heart rate reached during aerobic exercise which enables one's heartand lungs to receive the most benefit from a workout. This theoretical range varies based mostly on age; however, a person's physical condition, sex, and previous training also are used in the calculation. Below are two ways to calculate one's THR. In each of these methods, there is an element called "intensity" which is expressed as a percentage. The THR can be calculated as a range of 65–85% intensity. However, it is crucial to derive an accurate HRmax to ensure these calculations are meaningful.[citation needed]
Example for someone with a HRmax of 180 (age 40, estimating HRmax As 220 − age):
- 65% Intensity: (220 − (age = 40)) × 0.65 → 117 bpm
- 85% Intensity: (220 − (age = 40)) × 0.85 → 153 bpm
Karvonen method[edit]
The Karvonen method factors in resting heart rate (HRrest) to calculate target heart rate (THR), using a range of 50–85% intensity:[10]
- THR = ((HRmax − HRrest) × % intensity) + HRrest
Example for someone with a HRmax of 180 and a HRrest of 70:
- 50% Intensity: ((180 − 70) × 0.50) + 70 = 125 bpm
- 85% Intensity: ((180 − 70) × 0.85) + 70 = 163 bpm
Zoladz method[edit]
An alternative to the Karvonen method is the Zoladz method, which derives exercise zones by subtracting values from HRmax:
- THR = HRmax − Adjuster ± 5 bpm
- Zone 1 Adjuster = 50 bpm
- Zone 2 Adjuster = 40 bpm
- Zone 3 Adjuster = 30 bpm
- Zone 4 Adjuster = 20 bpm
- Zone 5 Adjuster = 10 bpm
Example for someone with a HRmax of 180:
- Zone 1(easy exercise): 180 − 50 ± 5 → 125 − 135 bpm
- Zone 4(tough exercise): 180 − 20 ± 5 → 155 − 165 bpm
Maximum heart rate
The maximum heart rate (HRmax) is the highest heart rate an individual can achieve without severe problems through exercise stress,[11][12] and generally decreases with age. Since HRmax varies by individual, the most accurate way of measuring any single person's HRmax is via a cardiac stress test. In this test, a person is subjected to controlled physiologic stress (generally by treadmill) while being monitored by an ECG. The intensity of exercise is periodically increased until certain changes in heart function are detected on the ECG monitor, at which point the subject is directed to stop. Typical duration of the test ranges ten to twenty minutes.
Adults who are beginning a new exercise regimen are often advised to perform this test only in the presence of medical staff due to risks associated with high heart rates. For general purposes, a formula is often employed to estimate a person's maximum heart rate. However, these predictive formulas have been criticized as inaccurate because they generalized population-averages and usually focus on a person's age. It is well-established that there is a "poor relationship between maximal heart rate and age" and large standard deviations around predicted heart rates.[13] (see Limitations of Estimation Formulas).
A number of formulas are used to estimate HRmax
Tanaka, Monahan, & Seals[edit]
From Tanaka, Monahan, & Seals (2001):
- HRmax = 208 − (0.7 × age) [14]
Their meta-analysis (of 351 prior studies involving 492 groups and 18,712 subjects) and laboratory study (of 514 healthy subjects) concluded that, using this equation, HRmax was very strongly correlated to age (r = −0.90). The regression equation that was obtained in the laboratory-based study (209 − 0.7 x age), was virtually identical to that of the meta-study. The results showed HRmax to be independent of gender and independent of wide variations in habitual physical activity levels. This study found a standard deviation of ~10 beats per minute for individuals of any age, meaning the HRmax formula given has an accuracy of ±20 beats per minute.[14]
In 2007, researchers at the Oakland University analyzed maximum heart rates of 132 individuals recorded yearly over 25 years, and produced a linear equation very similar to the Tanaka formula, HRmax = 206.9 − (0.67 × age), and a nonlinear equation, HRmax = 191.5 − (0.007 × age2). The linear equation had a confidence interval of ±5–8 bpm and the nonlinear equation had a tighter range of ±2–5 bpm. Also a third nonlinear equation was produced: HRmax = 163 + (1.16 × age) − (0.018 × age2).[15]
Haskell and Fox[edit]
Notwithstanding the research of Tanaka, Monahan, & Seals, the most widely cited formula for HRmax (which contains no reference to any standard deviation) is still:
- HRmax = 220 − age
Although attributed to various sources, it is widely thought to have been devised in 1970 by Dr. William Haskell and Dr. Samuel Fox.[16] Inquiry into the history of this formula reveals that it was not developed from original research, but resulted from observation based on data from approximately 11 references consisting of published research or unpublished scientific compilations.[17] It gained widespread use through being used byPolar Electro in its heart rate monitors,[16] which Dr. Haskell has "laughed about",[16] as the formula "was never supposed to be an absolute guide to rule people's training."[16]
While it is the most common (and easy to remember and calculate), this particular formula is not considered by reputable health and fitness professionals to be a good predictor of HRmax. Despite the widespread publication of this formula, research spanning two decades reveals its large inherent error, Sxy = 7–11 bpm. Consequently, the estimation calculated by HRmax = 220 − age has neither the accuracy nor the scientific merit for use in exercise physiology and related fields.[17]
Robergs and Landwehr
A 2002 study[17] of 43 different formulas for HRmax (including that of Haskell and Fox – see above) published in the Journal of Exercise Psychology concluded that:
- no "acceptable" formula currently existed (they used the term "acceptable" to mean acceptable for both prediction of VO2, and prescription of exercise training HR ranges)
- the least objectionable formula was:
-
- HRmax = 205.8 − (0.685 × age)
- This had a standard deviation that, although large (6.4 bpm), was considered acceptable for prescribing exercise training HR ranges.
Gulati (for women)
Research conducted at Northwestern University by Martha Gulati, et al., in 2010[18] suggested a maximum heart rate formula for women:
- HRmax = 206 − (0.88 × age)
Gellish
A 2008 study from Lund, Sweden gives reference values (obtained during bicycle ergometry) for men:
- HRmax = 203.7 / ( 1 + exp( 0.033 × (age − 104.3) ) ) [19]
and for women:
- HRmax = 190.2 / ( 1 + exp( 0.0453 × (age − 107.5) ) ) [20]
Other formulae
- HRmax = 206.3 − (0.711 × age)
-
- (Often attributed to "Londeree and Moeschberger from the University of Missouri")
- HRmax = 217 − (0.85 × age)
-
- (Often attributed to "Miller et al. from Indiana University")
Limitations
Maximum heart rates vary significantly between individuals.[16] Even within a single elite sports team, such as Olympic rowers in their 20s, maximum heart rates have been reported as varying from 160 to 220.[16] Such a variation would equate to a 60 or 90 year age gap in the linear equations above, and would seem to indicate the extreme variation about these average figures.
Figures are generally considered averages, and depend greatly on individual physiology and fitness. For example, an endurance runner's rates will typically be lower due to the increased size of the heart required to support the exercise, while a sprinter's rates will be higher due to the improved response time and short duration. While each may have predicted heart rates of 180 (= 220 − age), these two people could have actual HRmax 20 beats apart (e.g., 170-190).
Further, note that individuals of the same age, the same training, in the same sport, on the same team, can have actual HRmax 60 bpm apart (160–220):[16] the range is extremely broad, and some say "The heart rate is probably the least important variable in comparing athletes."[16]
Heart rate reserve
Heart rate reserve (HRreserve) is the difference between a person's measured or predicted maximum heart rate and resting heart rate. Some methods of measurement of exercise intensity measure percentage of heart rate reserve. Additionally, as a person increases their cardiovascular fitness, their HRrest will drop, that the heart rate reserve will increase. Percentage of HRreserve is equivalent to percentage of VO2 reserve.[21]
- HRreserve = HRmax − HRrest
This is often used to gauge exercise intensity (first used in 1957 by Karvonen).[22]
Karvonen's study findings have been questioned, due to the following:
- The study did not use VO2 data to develop the equation.
- Only six subjects were used, and the correlation between the percentages of HRreserve and VO2 max was not statistically significant.[23]
Heart rate recovery
Heart rate recovery (HRrecovery) is the reduction in heart rate at peak exercise and the rate as measured after a cool-down period of fixed duration.[24] A greater reduction in heart rate after exercise during the reference period is associated with a higher level of cardiac fitness.[25]
Heart rates that do not drop by more than 12 bpm one minute after stopping exercise are associated with an increased risk of death.[24] Investigators of the Lipid Research Clinics Prevalence Study, which included 5,000 subjects, found that patients with an abnormal HRrecovery (defined as a decrease of 42 beats per minutes or less at two minutes post-exercise) had a mortality rate 2.5 times greater than patients with a normal recovery.[25] Another study by Nishime et al. and featuring 9,454 patients followed for a median period of 5.2 years found a four-fold increase in mortality in subjects with an abnormal HRrecovery (≤12 bpm reduction one minute after the cessation of exercise).[25] Shetler et al. studied 2,193 patients for thirteen years and found that a HRrecovery of ≤22 bpm after two minutes "best identified high-risk patients".[25] They also found that while HRrecovery had significant prognostic value it had no diagnostic value.[25]
Development
See also: Heart development
The human heart beats more than 3.5 billion times in an average lifetime.
The heartbeat of a human embryo begins at approximately 21 days after conception, or five weeks after the last normal menstrual period (LMP), which is the date normally used to date pregnancy in the medical community. The electrical depolarizations that trigger cardiac myocytes to contract arise spontaneously within the myocyteitself. The heartbeat is initiated in the pacemaker regions and spreads to the rest of the heart through a conduction pathway. Pacemaker cells develop in the primitive atrium and the sinus venosus to form thesinoatrial node and the atrioventricular node respectively. Conductive cells develop the bundle of His and carry the depolarization into the lower heart.
The human heart begins beating at a rate near the mother’s, about 75-80 beats per minute (BPM). The embryonic heart rate then accelerates linearly for the first month of beating, peaking at 165-185 BPM during the early 7th week, (early 9th week after the LMP). This acceleration is approximately 3.3 BPM per day, or about 10 BPM every three days, an increase of 100 BPM in the first month.[26]
After peaking at about 9.2 weeks after the LMP, it decelerates to about 150 BPM (+/-25 BPM) during the 15th week after the LMP. After the 15th week the deceleration slows reaching an average rate of about 145 (+/-25 BPM) BPM at term. The regression formula which describes this acceleration before the embryo reaches 25 mm in crown-rump length or 9.2 LMP weeks is:

There is no difference in male and female heart rates before birth.[27]
Clinical significance
Measurement
Manual measurement
Heart rate is measured by finding the pulse of the heart. This pulse rate can be found at any point on the body where the artery's pulsation is transmitted to the surface by pressuring it with the index and middle fingers; often it is compressed against an underlying structure like bone. (A good area is on the neck, under the corner of the jaw.) The thumb should not be used for measuring another person's heart rate, as its strong pulse may interfere with the correct perception of the target pulse.
The radial artery is the easiest to use to check the heart rate. However, in emergency situations the most reliable arteries to measure heart rate are carotid arteries. This is important mainly in patients with atrial fibrillation, in whom heart beats are irregular and stroke volume is largely different from one beat to another. In those beats following a shorter diastolic interval left ventricle doesn't fill properly, stroke volume is lower and pulse wave is not strong enough to be detected by palpation on a distal artery like the radial artery. It can be detected, however, by doppler.[28][29]
Possible points for measuring the heart rate are:
- The ventral aspect of the wrist on the side of the thumb (radial artery).
- The ulnar artery.
- The neck (carotid artery).
- The inside of the elbow, or under the biceps muscle (brachial artery).
- The groin (femoral artery).
- Behind the medial malleolus on the feet (posterior tibial artery).
- Middle of dorsum of the foot (dorsalis pedis).
- Behind the knee (popliteal artery).
- Over the abdomen (abdominal aorta).
- The chest (apex of the heart), which can be felt with one's hand or fingers. It is also possible to auscultate the heart using a stethoscope.
- The temple (superficial temporal artery).
- The lateral edge of the mandible (facial artery).
- The side of the head near the ear (posterior auricular artery).
Electronic measurement
A more precise method of determining heart rate involves the use of an electrocardiograph, or ECG (also abbreviatedEKG). An ECG generates a pattern based on electrical activity of the heart, which closely follows heart function. Continuous ECG monitoring is routinely done in many clinical settings, especially in critical care medicine. On the ECG, instantaneous heart rate is calculated using the R wave-to-R wave (RR) interval and multiplying/dividing in order to derive heart rate in heartbeats/min. Multiple methods exist:
- HR = 1,500/(RR interval in millimeters)
- HR = 60/(RR interval in seconds)
- HR = 300/number of "large" squares between successive R waves.
Heart rate monitors allow measurements to be taken continuously and can be used during exercise when manual measurement would be difficult or impossible (such as when the hands are being used). Various commercial heart rate monitors are also available. Some monitors, used during sport, consist of a chest strap with electrodes. The signal is transmitted to a wrist receiver for display.
Alternative methods of measurement include pulse oximetry and seismocardiography.[30]
Tachycardia
Tachycardia is a resting heart rate more than 100 beats per minute. This number can vary as smaller people and children have faster heart rates than average adults.
Physiological conditions where tachycardia occurs:
- Exercise
- Pregnancy
- Emotional conditions such as anxiety or stress.
Pathological conditions where tachycardia occurs:
- Sepsis
- Fever
- Anemia
- Hypoxia
- Hyperthyroidism
- Hypersecretion of catecholamines
- Cardiomyopathy
- Valvular heart diseases
- Acute Radiation Syndrome
Bradycardia
Bradycardia was defined as a heart rate less than 60 beats per minute when textbooks asserted that the normal range for heart rates was 60–100 BPM. The normal range has since been revised in textbooks to 50–90 BPM for a human at total rest. Setting a lower threshold for bradycardia prevents misclassification of fit individuals as having a pathologic heart rate. The normal heart rate number can vary as children and adolescents tend to have faster heart rates than average adults. Bradycardia may be associated with medical conditions such as hypothyroidism.
Trained athletes tend to have slow resting heart rates, and resting bradycardia in athletes should not be considered abnormal if the individual has no symptoms associated with it. For example, Miguel Indurain, a Spanish cyclist and five time Tour de France winner, had a resting heart rate of 28 beats per minute,[31] one of the lowest ever recorded in a healthy human. Daniel Green achieved the world record for the slowest heartbeat in a healthy human with a heart rate of just 26 bpm in 2014.[32]
Arrhythmia
Arrhythmias are abnormalities of the heart rate and rhythm (sometimes felt as palpitations). They can be divided into two broad categories: fast and slow heart rates. Some cause few or minimal symptoms. Others produce more serious symptoms of lightheadedness, dizziness and fainting.
Correlation with cardiovascular mortality risk
A number of investigations indicate that faster resting heart rate has emerged as a new risk factor for mortality in homeothermic mammals, particularly cardiovascular mortality in human beings. Faster heart rate may accompany increased production of inflammation molecules and increased production of reactive oxygen species in cardiovascular system, in addition to increased mechanical stress to the heart. There is a correlation between increased resting rate and cardiovascular risk. This is not seen to be "using an allotment of heart beats" but rather an increased risk to the system from the increased rate.[33]
An Australian-led international study of patients with cardiovascular disease has shown that heart beat rate is a key indicator for the risk of heart attack. The study, published in The Lancet (September 2008) studied 11,000 people, across 33 countries, who were being treated for heart problems. Those patients whose heart rate was above 70 beats per minute had significantly higher incidence of heart attacks, hospital admissions and the need for surgery. University of Sydney professor of cardiology Ben Freedman from Sydney's Concord hospital, said "If you have a high heart rate there was an increase in heart attack, there was about a 46 percent increase in hospitalizations for non-fatal or fatal heart attack."[34]
Standard textbooks of physiology and medicine mention that heart rate (HR) is readily calculated from the ECG as follows:
- HR = 1,500/RR interval in millimeters, HR = 60/RR interval in seconds, or HR = 300/number of large squares between successive R waves. In each case, the authors are actually referring to instantaneous HR, which is the number of times the heart would beat if successive RR intervals were constant. However, because the above formula is almost always mentioned, students determine HR this way without looking at the ECG any further.
Very low heart rate (bradycardia) may be associated with heart block.[medical citation needed] It may also arise from autonomous nervous system impairment.[medical citation needed]
What should you know about your heart rate?
Even if you’re not an athlete, knowledge about your heart rate can help you monitor your fitness level — and it might even help you spot developing health problems.
Your heart rate, or pulse, is the number of times your heart beats per minute. Normal heart rate varies from person to person. Knowing yours can be an important heart-health gauge.
“As you age, changes in the rate and regularity of your pulse can change and may signify a heart condition or other condition that needs to be addressed,” said Richard Stein, M.D., professor of medicine and cardiology at the New York University School of Medicine in New York City and a volunteer for the American Heart Association.
Where is it and what is a normal heart rate?
The best places to find your pulse are the:
- wrists
- inside of your elbow
- side of your neck
- top of the foot
To get the most accurate reading, put your finger over your pulse and count the number of beats in 60 seconds, Stein said.
Your resting heart rate is the heart pumping the lowest amount of blood you need because you’re not exercising. If you’re sitting or lying and you’re calm, relaxed and aren’t ill, your heart rate is normally between 60 (beats per minute) and 100 (beats per minute), Stein said.
But a heart rate lower than 60 doesn’t necessarily signal a medical problem. It could be the result of taking a drug such as a beta blocker. A lower heart rate is also common for people who get a lot of physical activity or are very athletic, Stein said. Active people often have lower heart rates because their heart muscle is in better condition and doesn’t need to work as hard to maintain a steady beat.
“Moderate physical activity doesn’t usually change the resting pulse much,” Stein said. “If you’re very fit, it could change to 40. A less active person might have a heart rate between 60 and 100,” he added. That’s because the heart muscle has to work harder to maintain bodily functions, making it higher.
How Other Factors Affect Heart Rate
- Air temperature: When temperatures (and the humidity) soar, the heart pumps a little more blood, so your pulse rate may increase, but usually no more than five to 10 beats a minute.
- Body position: Resting, sitting or standing, your pulse is usually the same. Sometimes as you stand for the first 15 to 20 seconds, your pulse may go up a little bit, but after a couple of minutes it should settle down. Emotions: If you’re stressed, anxious or “extraordinarily happy or sad” your emotions can raise your pulse.
- Body size: Body size usually doesn’t usually change pulse. If you’re very obese, you might see a higher resting pulse than normal, but usually not more than 100.
- Medication use: Meds that block your adrenaline (beta blockers) tend to slow your pulse, while too much thyroid medication or too high of a dosage will raise it.
When To Call Your Doctor
If you’re on a beta blocker to decrease your heart rate (and lower blood pressure) or to control a another abnormal rhythm (arrhythmia), your doctor may ask you to monitor and log your heart rate. Keeping tabs on your heart rate can help your doctor determine whether to change the dosage or switch to a different medication.
“If your pulse is very low or if you have frequent episodes of unexplained fast heart rates, especially if they cause you to feel weak or dizzy or faint, tell your doctor, who can decide if it’s an emergency,” Stein said. “Your pulse is one tool to help get a picture of your health.
Heart rate, also known as pulse, is the number of times a person's heart beats per minute. A normal heart rate depends on the individual, age, body size, heart conditions, whether the person is sitting or moving, medication use and even air temperature. Even emotions can have an impact on heart rate. For example, getting excited or scared can increase the heart rate. But most importantly, getting fitter lowers the heart rate, by making heart muscles work more efficiently.
"Your heart is a muscle and just like strengthening other muscles by doing activities, you can do the same thing with your heart," said Dr. Mary Ann Bauman, an internist at Integris Baptist Medical Center in Oklahoma City.
Knowledge about your heart rate can help you monitor your fitness level, and it may help you spot developing health problems if you are experiencing other symptoms.
"If you are an athlete and you're training, or if you are having symptoms such as dizziness, then knowing your heart rate is important," Bauman said. "But as a general rule, unless somebody is having problems, it's not very important to always know what your heart rate is."
How to measure heart rate
The easiest places to measure your heart rate are on the wrists or one side of the neck. For an accurate reading, put two fingers over one of these areas and count the number of beats in 60 seconds. You can also do this for 20 seconds and multiply by three, which may be easier, Bauman said. Using your thumb may be confusing because sometimes you can feel a pulse in the thumb, she said.
Resting heart rate
Your resting heart rate is your pulse when you are calmly sitting or lying. It’s best to measure your resting heart rate it in the morning before you get out of bed, according to the American Heart Association (AHA). For adults 18 and older, a normal resting heart rate is between 60 and 100 beats per minute (bpm), depending on the person’s physical condition and age. For children ages 6 to 15, the normal resting heart rate is between 70 and 100 bpm, according to the AHA.
But a heart rate lower than 60 doesn’t necessarily mean you have a medical problem. Active people often have lower heart rates because their heart muscles don't need to work as hard to maintain a steady beat. Athletes and people who are very fit can have resting heat rate of 40 bpm.
A resting heart rate lower than 60 could also be the result of taking certain medications. "Many medications people take especially medication for blood pressure, such as the beta blockers, will lower your heart rate," Bauman said.
If coupled with symptoms, a low heart rate may signal a problem.
"A low heart rate in somebody who is having dizziness and lightheadedness may indicate that they have an abnormality that needs to be looked at," Bauman said.
Maximum and target heart rate
There is no definitive medical advice on when a resting heart rate is too high, but most medical experts agree that a consistent heart rate in the upper levels can put too much stress on the heart and other organs. If a person has a high heart rate at rest and is experiencing other symptoms, doctors may examine his or her heart function, Bauman said.
Knowing your heart rate during workout sessions can help know whether you are doing too much or not enough, the AHA says. When people exercise in their "target heart zone," they gain the most benefits and improve their heart's health. When your heart rate is in the target zone you know "you are pushing the muscle to get stronger," Bauman said.
A person's target heart rate zone is between 50 percent and 85 percent of his or her maximum heart rate, according to the AHA.
Most commonly, maximum heart rate is calculate by subtracting your age from 220:
- 220 - Age. For a 30-year-old person, for example: 220 - 30 = 190.
The target zone for a 30-year-old person would be between 50 and 85 percent of his or her maximum heart rate:
- 50 percent level: 190 x 0.50 = 95 bpm
- 85 percent level: 190 x 0.85 = 162 bpm
The formula for maximum heart rate works well for people under 40 but for older people it may overestimate their maximum heart rate, Bauman said. For older people, a better formula for the maximum heart rate is:
- 208 – (0.75 x Age)
You can either manually calculate your heart rate during exercise or use heart rate monitors that wrap around the chest, or are included in sports watches. [Related: The Best Heart Rate Monitor Watches for Exercise]
However, that's not to say that exercising without getting the heart rate up to the target zone has no benefit, Bauman said.
"So many people just aren't doing any exercise that I worry less about them reaching their target heart rate and more about them getting out and moving their body," Bauman said.
Lowering a rapid heart rate
Pulse rates can spike due to nervousness, stress, dehydration and overexertion. Sitting down and taking slow, deep breaths can generally lower your heart rate. Exercising and getting fitter will usually lower heart rate, too.
Arrhythmia, tachycardia and other conditions
A number of conditions can affect your heart rate. An arrhythmia causes the heart to beat too fast, too slow or with an irregular rhythm.
Tachycardia is generally considered to be a resting heart rate of over 100 beats per minute, according to the National Institutes of Health, and generally caused when electrical signals in the heart's upper chambers fire abnormally. If the heart rate is closer to 150 bpm or higher, it is a condition known as supraventricular tachycardia (SVT). In SVT, your heart’s electrical system, which controls the heart rate, is out of whack. This generally requires medical attention.
Bradycardia is a condition where the heart rate is too low, typically less than 60 bpm. This can be the result of problems with the sinoatrial node, which acts as the pacemaker, or damage to the heart as a result of a heart attack or cardiovascular disease.
High blood pressure vs. high heart rate
Some people confuse high blood pressure with a high heart rate. Blood pressure is the measurement of the force of the blood against the walls of arteries, while pulse rate is the number of times your heart beats per minute.
There is no direct correlation between the two, and high blood pressure does not necessarily result in a high pulse rate, and vice versa. Heart rate goes up during strenuous activity, but a vigorous workout may only modestly increase blood pressure.
How to Read an ECG Strip
ECG paper is a grid where time is measured along the horizontal axis.
- Each small square is 1 mm in length and represents 0.04 seconds.
- Each larger square is 5 mm in length and represents 0.2 seconds.
Voltage is measured along the vertical axis.
- 10 mm is equal to 1mV in voltage.
- The diagram below illustrates the configuration of ECG graph paper and where to measure the components of the ECG wave form
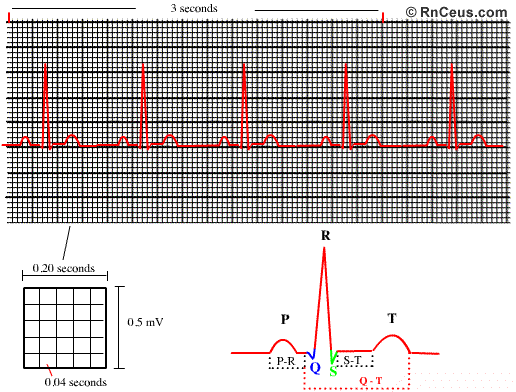
Heart rate can be easily calculated from the ECG strip:
- When the rhythm is regular, the heart rate is 300 divided by the number of large squares between the QRS complexes.
- For example, if there are 4 large squares between regular QRS complexes, the heart rate is 75 (300/4=75).
- The second method can be used with an irregular rhythm to estimate the rate. Count the number of R waves in a 6 second strip and multiply by 10.
- For example, if there are 7 R waves in a 6 second strip, the heart rate is 70 (7x10=70).
Determining Heart Rate
There are two different rates that can be determined on ECGs. The atrial rate is indicated by the frequency of the P waves and the ventricular rate is indicated by the frequency of the QRS complexes.
Normally, the atrial rate should be the same as the ventricular rate in the absence of disease, however certain conditions, such as third degree AV nodal block or ventricular tachycardia can alter this normal relationship causing “AV dissociation”. In this setting, the atrial rate (P waves) and ventricular rate (QRS complexes) are at different heart rates.
One quick and easy way to measure the ventricular rate is to examine the RR interval (distance between two consecutive R waves) and use a standard scale to find the rate. If two consecutive R waves are separated by only one large box, then the rate is 300 beats per minute. If the R waves are separated by two large blocks, then the ventricular rate is 150 beats per minute. The scale continues down to show that if two consecutive R waves are separated by 8 large boxes, then the rate is 37 beats per minute. The pictorial explanation of this method is to the right.
Another quick way to calculate the rate is based on the fact that the entire ECG is 10 seconds. So by counting the number of QRS complexes and multiplying by 6, the number per minute can be calculated (since 10 seconds times 6 is 60 seconds or 1 minute). This is a better method when the QRS complexes are irregular (such as during atrial fibrillation) which makes the first method less accurate, since the RR intervals may vary from beat to beat in this setting. Below are some examples using each method.
Example 1: Note that the QRS complexes are about five and a half large boxes apart. Referencing the above image it can be determined that the ventricular heart rate is between 50 and 60 beats per minute. This is a full 10 second rhythm strip. There are 9 QRS complexes total. Multiply the number of QRS complexes by 6 and the exact heart rate is 54 beats per minute. There is 1 P wave for each QRS complex and thus the atrial rate is the same.

Example 2: These QRS complexes are exactly three large boxes apart and thus the ventricular heart rate is 100 beats per minute. Now multiple the number of QRS complexes on this strip by 6. This would be 17 x 6 = 102. There is 1 P wave for each QRS complex and thus the atrial rate is the same.

Example 3: These QRS complexes are less than 2 large boxes apart and thus the heart rate is between 150 and 300. Multiplying the number of QRS complexes by 6 would give 29 x 6 = 174 beats per minute. There is likely 1 P wave for each QRS complex (difficult to see on this strop) and thus the atrial rate is likely the same.

Example 4: The below ECG strip shows the irregularly irregular QRS complexes present during atrial fibrillation. Using the first method to determine heart rate would NOT be accurate since the R-R intervals vary significantly. The best way to determine the ventricular heart rate would be to simply count the QRS complexes and multiple by 6 which would be 15 x 6 = 90 beats per minute. The P waves are not able to be identified in atrial fibrillation and it is assumed that the atrial rate is between 400-600 beats per minute.

Example 5: This ECG strip shows "AV dissociation" meaning the P waves (indicating atrial activity) are at a different rate than the QRS complexes (indicating ventricular activity). This rhythm is actually an accelerated idioventricular rhythm (slow ventricular tachycardia). The atrial rate is indicated by the P waves. There are almost exactly 5 large boxes between P waves indicating an atrial rate of 60 beats per minute. There are a total of 10 P waves on this strip (difficult to see some of them as they are intermittently buried in the QRS complexes) and 10 x 6 = 60 confirming the first method. There are just more than 4 big boxes between each QRS complexes and thus the ventricular rate is between 60 and 75. Since there is a total of 11 QRS complexes in this full 10 second strip, the actual ventricular rate is 11 x 6 = 66 beats per minute.

Determining Rhythm
The rhythm is either sinus rhythm or not sinus rhythm. Sinus rhythm refers to the origination of the electrical activity coming from the sinus node (SA node or sinoatrial node). This results in an upright P wave in lead II on the ECG.
If there is a P wave before every QRS complex and it has a "sinus morphology", then “normal sinus rhythm” or “NSR” is said to be present. A sinus morphology is an upright P wave in lead II and biphasic (up and down) in lead V1. The first ECG strip below shows a P wave with sinus morphology and thus normal sinus rhythm. If the P wave has a different morphology than the typical sinus morphology, then it is termed "ectopic" simply meaning coming from somewhere other than the sinus node. Ectopic atrial rhythms (including atrial tachycardia), multifocal atrial tachycardia and junctional rhythms all have P waves that are not of sinus morphology and will be reviewed in detail later. The second strip below shows an ectopic atrial rhythm. Note that the P wave is down in lead II and only up (not biphasic) in lead V1.

If there is sinus rhythm and the heart rate is greater than 100, then “sinus tachycardia” is present. If the there is sinus rhythm and the heart rate is less than 60, then “sinus bradycardia” is present. Below are an example of each of these:
If there are no P waves present or the P wave morphology is not normal, then the exact rhythm must be determined. Multiple other arrhythmias exist and include atrial fibrillation, atrial flutter, and ventricular rhythms such as ventricular tachycardia or ventricular fibrillation. These are discussed in detail in the ECG criteria review sections.
Here are three more examples of rhythms other than sinus rhythm:

Note that when “AV dissociation” is present as previously described (complete heart block or ventricular tachycardia), there may not be a P wave before every QRS complex, however as long as the P wave is upright in lead II, sinus rhythm is still said to be present.
Determining ECG Axis
The axis of the ECG is the major direction of the overall electrical activity of the heart. It can be normal, leftward (left axis deviation or LAD), rightward (right axis deviation or RAD), or indeterminate (northwest axis). The QRS axis is the most important to determine, however the P wave or T wave axis can also be measured.
To determine the QRS axis, the limb leads need to be examined (not the precordial leads). The depiction of the standard leads and their relationship to the cardiac axis is below.

Note that lead I is at zero degrees, lead II is at +60 degrees, and lead III is at +120 degrees. Lead aVL (L for left arm) is at -30 degrees, lead aVF (F for foot) is at +90 degrees, and the negative of lead aVR (R for right arm) is at +30 degrees. The positive of lead aVR is actually at -150 degrees.
Memorizing the above picture is crucial to accurately determining axis, however there are some shortcuts to quickly determine the axis as mentioned below.
The normal QRS axis should be between - 30 and +90 degrees. Left axis deviation is defined as the major QRS vector falling between -30 and -90 degrees. Right axis deviation occurs with the QRS axis is between +90 and +180 degrees. Indeterminate axis is between +/- 180 and -90 degrees. This is summarized in the image below:

LAD = Left Axis Deviation
RAD = Right Axis Deviation
NW = northwest axis or indeterminate axis
The fastest, non-specific method to determine the QRS axis is to find the major direction of the QRS complex (positive or negative) in leads I and aVF.
Normal QRS Axis
If the QRS complex is upright (positive) in both lead I and lead aVF, then the axis is normal. The below image demonstrates this example with the electrical vector heading towards the positive of lead I and the positive of lead aVF as indicated by the arrows. The QRS axis is thus between these two arrows which falls within the normal range.

Left Axis Deviation
If the QRS is upright in lead I (positive) and downward in lead aVF (negative), then the axis is between 0 and -90 degrees. However recall that left axis deviation is defined as between -30 and -90 and thus this scenario is not always technically left axis deviation. In this scenario, the QRS axis could fall between 0 and -30 which is within normal limits. To further distinguish normal from left axis deviation in this setting, look at lead II. If lead II is downward (negative), then the axis is more towards -120 and left axis deviation is present. If the QRS complex in lead II is upright (positive), then the axis is more towards +60 degrees and the QRS axis is normal.
Causes of left axis deviation (LAD) are below. Note that the first 3 account for almost 90% of ECG tracings with left axis deviation.
1. Normal variant
3. Left ventricular hypertrophy (rarely, usually axis normal with LVH)
4. Left bundle branch block (rarely)
5. Mechanical shift of heart in the chest (lung disease, prior chest surgery etc...)
7. Wolff-Parkinson-White syndrome with "pseudoinfarct" pattern
8. Ventricular rhythms (accelerated idioventricular or ventricular tachycardia)
9. Ostium primum atrial septal defect
Below is an example of left axis deviation to help visualize the above explanation:

Right Axis Deviation
If the QRS is predominantly negative in lead I and positive in lead aVF, then the axis is rightward (right axis deviation). Causes of right axis deviation include:
1. Normal variation
5. Dextrocardia
6. Ventricular rhythms (accelerated idioventricular or ventricular tachycardia)
7. Lateral wall myocardial infarction
9. Acute right heart strain/pressure overload a.k.a. "McGinn-White Sign" or S1Q3T3 that occurs in pulmonary embolus.
Below is a pictorial example of right axis deviation:

Indeterminate Axis
If the QRS is downward (negative) in lead I and downward (negative) in lead aVF, then the axis is indeterminate and sometimes referred to as "northwestern axis". This finding is uncommon and usually from ventricular rhythms, but can also be from paced rhythms, lead misplacement and certain congenital heart diseases.

P Wave

P wave: Indicates atrial depolarization
The P wave occurs when the SA node (sinus node or sinoatrial node) creates an action potential that depolarizes the atria. The P wave should be upright in lead II if the action potential is originating from the SA node. In this setting, the ECG is said to demonstrate a "normal sinus rhythm" abbreviated "NSR". As long as the atrial depolarization is able to spread through the AV node to the ventricles, each P wave should be followed by a QRS complex.
Multiple abnormalities of the P wave exist and are discussed in detail in the ECG criteria review sections. Atrial enlargements can widen the P wave or increase the P wave amplitude. Ectopic atrial rhythms can alter the normal morphology of the P waves. There are many heart rhythms in which the P waves are not able to be identified such as atrial fibrillation and sometimes junctional rhythms. At times, the P waves can be buried at the end of the QRS complex causing a “short RP” scenario such as seen in atrioventricular reentry tachycardia (AVNRT).
PR Interval
The time from the beginning of the P wave (atrial depolarization) to the beginning of the QRS complex (ventricular depolarization) is the PR interval. This represents the time that it takes for the electrical impulse generated in the sinus node to travel through the atria and across the AV node to the ventricles. The normal PR interval is 0.12 to 0.20 seconds or 120 to 200 milliseconds (ms).

Multiple abnormalities of the PR interval can occur including prolongation (as depicted on the right), shortening of the PR interval and variation from beat to beat. These are discussed in detail in the ECG criteria review sections. A prolonged PR interval indicates delayed conduction of the SA nodal impulse to the ventricles and is called 1st degree AV block. A short PR interval can be seen when the AV node delay is bypassed such as in Wolff-Parkinson-White syndrome or Lown-Ganong-Levine syndrome. The PR interval will vary in seconds degree type I AV block (Wenckebach).
Note that the PR interval can be altered by changing sympathetic and parasympathetic tone. Because of this, medications such as beta-blockers can lengthen the PR interval causing a first degree AV block. Also, a longer PR interval can cause the first heart sound on examination to sound soft and vise versa.
PR Segment
The PR segment is the portion of the ECG from the end of the P wave to the beginning of the QRS complex. The PR segment is different than the PR interval which is measured in units of time (ms).
Remember that segments are different than intervals. The important factor to analyze in segments on the ECG is their change from the isoelectric line (elevation or depression) while the important thing to analyze for intervals is their duration.
Abnormalities of the PR segment are not very common, however can indicated certain cardiac disease states. PR segment depression can indicate pericarditis or atrial infarction. PR segment elevation occurs in lead aVR in the setting of pericarditis.
Q Wave
The Q wave is the first downward deflection after the P wave and is the first element in the QRS complex. When the first deflection of the QRS complex is upright, then no Q wave is present. The normal individual will have a small Q wave in many, but not all ECG leads.

Abnormalities of the Q waves are mostly indicative of myocardial infarctions and are discussed in the ECG criteria review sections. The terms Q wave myocardial infarction and non-Q wave myocardial infarction are old designations of different types of myocardial infarctions where the end result is Q wave development or the absence of Q wave development respectively. ECG examples of different Q wave patterns are below:
- Old Inferior Wall MI ECG Example 1
- Old Inferior Wall MI ECG Example 2
- Normal inferior Q waves - NOT old inferior MI ECG Exmaple
- Old Anterior MI ECG Example 1
R Wave
The R wave is the first upward deflection after the P wave and is part of the QRS complex. The R wave morphology itself is not of great clinical importance, however at times it can vary. In lead V1, the R wave should be small. The R wave becomes larger throughout the precordial leads (V1 to V6) to the point where the R wave is larger than the S wave in lead V4. The S wave then becomes quite small in lead V6. This is called normal "R wave progression". When the R wave remains small in leads V3-V4 (smaller than the S wave), the term "poor R wave progression" is used and is depicted below:
Remember, in lead V1 the R wave is usually quite small, however if the R wave is large in V1 (greater in amplitude than the S wave), then significant pathology may be present. Causes of an R wave to S wave ratio of greater than 1 in lead V1 include a right bundle branch block, Wolff-Parkinson-White syndrome, an acute posterior myocardial infarction, right ventricular hypertrophy and isolated posterior wall hypertrophy (which can occur in Duchenne's muscular dystrophy).
In the setting of a right bundle branch block there may be two R waves present giving the classic “bunny ear” appearance of the QRS complex. In this setting, the second R wave is termed R’ or “R prime”.
S Wave
The S wave is the first downward deflection of the QRS complex that occurs after the R wave.

An S wave may not be present in all ECG leads in a given patient. In the normal ECG, there is a large S wave in V1 which progressively becomes smaller to the point where almost no S wave is present in V6. A large slurred S wave is seen in lead I and V6 in the setting of a right bundle branch block.
The presence or absence of the S wave does not have major clinical significance. Rarely is the morphology of the S wave discussed. In the setting of a pulmonary embolism, a large S wave may be present in lead I (part of the S1Q3T3 pattern seen in this disease state). At times the morphology of the S wave is examined to determine if ventricular tachycardia or supraventricular tachycardia with aberrancy is present. This is discussed elsewhere.
QRS Complex
The “QRS complex” is the combination of the Q wave, R wave and S wave and represents ventricular depolarization. This term can be confusing since not all ECG leads contain all three of these waves, yet a “QRS complex” is said to be present regardless. For example, the normal QRS complex in lead V1 does not contain a Q wave, but only a R wave and S wave, yet the combination of the R wave and S wave is still referred to as the QRS complex for this lead.

The normal duration (interval) of the QRS complex is 0.08 and 0.10 seconds (80 and 100 ms). When the duration is between 0.10 and 0.12 seconds it is intermediate or slightly prolonged. A QRS duration of greater than 0.12 seconds is considered abnormal.
The QRS duration will lengthen when electrical activity takes a long time to travel throughout the ventricular myocardium. The normal conduction system in the ventricles is called the His-Purkinje system and consists of cells that can conduct electricity quite rapidly. Thus, normal conduction of an electrical impulse through the AV node then to the ventricles via the His-Purkinje system is fast causing a normal QRS duration. When electrical activity does not conduct through the His-Purkinje system, but instead travels from myocyte to myocyte, a longer time is necessary and the QRS duration is widened.
A widened QRS duration occurs in the setting of a right bundle branch block, left bundle branch block, non-specific intraventricular conduction delay and during ventricular arrhythmias such as ventricular tachycardia all of which are discussed in detail in the ECG criteria review sections.
T Wave
The T wave occurs after the QRS complex and is a result of ventricular repolarization. T waves should be upright in most leads (except aVR and V1). T waves should be asymmetric in nature. The second portion of the T wave should have a steeper decline when compared to the incline of the first part of the T wave. If the T wave appears symmetric, cardiac pathology may be present such as ischemia.
Many abnormal T wave patterns exist which are reviewed in more detail in the ECG criteria review sections. These include hyperkalemia, Wellens syndrome, left ventricular hypertrophy with repolarization abnormalities, pericarditis (stage III), arrhythmogenic right ventricular dysplasia (ARVD) and hyperacute T waves during myocardial infarction.
QT Interval
The QT interval is the time from the beginning of the QRS complex (ventricular depolarization) to the end of the T wave (ventricular repolarization).
The normal QT interval is controversial and multiple normal durations have been reported. In general, the normal QT interval is less than 400-440 ms (0.4 to 0.44 seconds). Females have a longer QT interval than males. Also, lower heart rates result in a longer QT interval. A quick way to see if the QT interval is prolonged is to examine if the T wave ends past the half-way point between the R-R interval. If the T wave ends past the half-way point of the R-R interval, then it is prolonged.
Due to the heart rates effects, the corrected QT interval (QTc) is frequently used. The QTc is considered prolonged if greater than 450 ms in males and 470 in females and is calculated using the Bazett’s formula as below:

Prolongation of the QT interval can occur from multiple medications, electrolyte abnormalities (hypocalcemia, hypomagnesemia and hypokalemia) and certain disease states (i.e. intracranial hemorrhage).
ST Segment
The ST segment is the portion of the ECG from the end of the QRS complex to the beginning of the T wave. The ST segment normally remains isoelectric and thus ST segment depression or ST segment elevation can indicate cardiac pathology.
The ST segment is scrutinized on the ECG for the detection of myocardial ischemia. This can be done in the setting of exercise or pharmacologic stress testing as well.
Abnormal ST segments are reviewed based on the cause in the ECG criteria review sections and include anterior, posterior and inferior myocardial infarctions, left ventricular hypertrophy, pericarditis and Brugada syndrome.
TP Segment
The portion of the ECG from the end of the T wave to the beginning of the P wave. This segment should always be at baseline and is used as a reference to see if the ST segment is elevated or depressed since there are no specific disease conditions which elevate or depress the TP segment.
During states of tachycardia the TP segment is shortened and may be difficult to visualize altogether. It is good to examine the TP segment closely for the presence of U waves or atrial activity that could indicate pathology.
Sumber : Ragam informasi tentang Jantung dari internet.
My name is donald boykins , am here to appreciate Dr Akhigbe for using his herbal medicine to cure my Herpes virus. Is about 3 years and 6 months now I have been living with this virus and it has been a serious problem to me, I was so confused cause i have been taking several drugs to be cured but all of my effort was in vain,one morning I was browsing through the internet then I saw several testimonies about Dr. Akhigbe curing people from Herpes virus and immediately i contacted Dr. Akhigbe on his email: drrealakhigbe@gmail.com, i told him about my troubles and he told me that i must be cured, he gave me some instructions and which i rightly followed. so he prepared a herbal medicine and sent it to me which i used for 2 weeks and i was cured everything was like a dream to me and my Herpes virus was totally gone, dr .Akhigbe, God bless you and give you more power and ability for more cure.I don't know if there is any one out there suffering for herpes virus or any of these diseases..DIABETES, CANCER, HIV/AIDS, HERPES HEPATITiS A AND B. FEVER, EPILEPSY, MENINGITIS, DENGUE SCHIZOPHRENIA, PROSTATE, THYROID, BACTERIA, MALARIA, DICK AND BREAST ENLARGEMENT,MULTIPLE SCLEROSIS, TUBERCULOSIS, DIARRHEA, POLIO, ARTHMA, LUPUS, RABIS, JOINT PAIN, STOMACH PAIN,, HEART DISEASE, CHRONIC DISEASE,EPILEPSY, LUPUS, STROKE, SPINAL CORD INJURY, ECZEMA, KIDNEY DISEASE, ACME, BACK PAIN etc. why don't you contact dr.Akhigbe today and be free from your diseases because he is very good and honest Doctor and he is also called the godfather of herbal root contact him via email; drrealakhigbe@gmail.com or whatsApp him on +2348142454860
BalasHapuswebsite https:drrealakhigbe.weebly.com